How new magnets could accelerate climate action
Niron Magnetics aims to use cheap, widely available materials to make magnets for EVs and wind turbines.
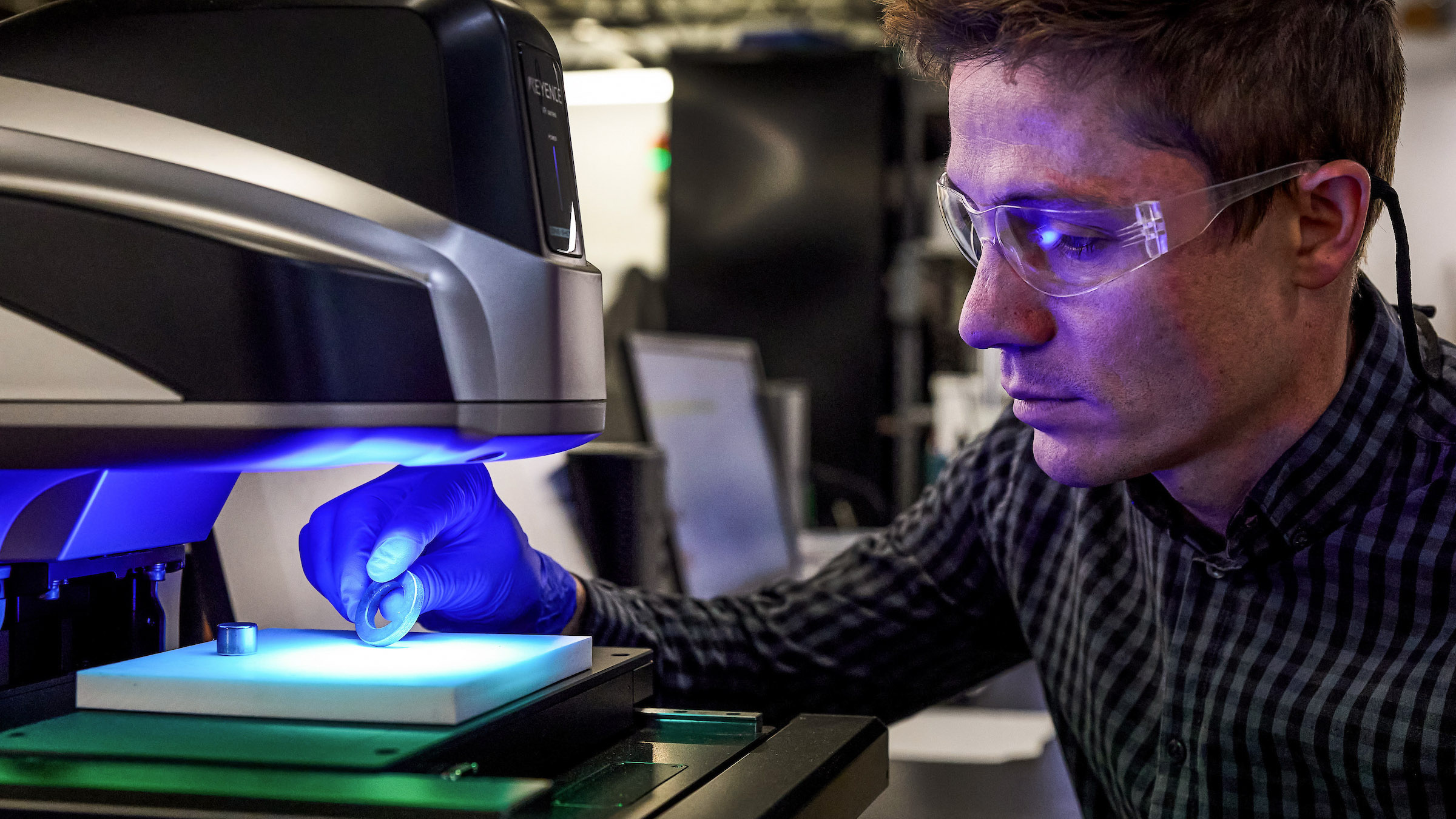
The motor in your vacuum cleaner and the one in your electric vehicle likely have at least one thing in common: they both rely on powerful permanent magnets to function. And the materials for those magnets could soon be in short supply.
Permanent magnets can maintain a magnetic field on their own without an electric charge. They’re commonly used in motors, making them spin when an electric field is applied. The permanent magnets used in high-end motors today are built using a class of materials called rare earth metals. Demand for these materials is expected to skyrocket in the coming decades, fueled in particular by the growth of electric vehicles and wind turbines. As mines and processing facilities struggle to keep up, supplies may stretch thin.
One Minnesota startup has been working to address this looming shortage. Niron Magnetics is building a large-scale manufacturing facility to produce iron nitride, a magnetic material derived from common elements, while also working to improve the material’s properties so that it can be used in stronger magnets to power more products. The results may help address yet another coming supply crunch that threatens to slow down action on climate change.
A growing gap
The permanent magnets you’re probably most familiar with are the cheap ones made from materials called ferrites that are holding up postcards and wedding announcements on your refrigerator.
But many of the devices sprinkled through our daily lives, like our vacuums and EVs, require much higher-powered magnets. Motors that generate motion using permanent magnets tend to be more powerful and efficient, so rare earth metals, such as neodymium and dysprosium, have become vital for a wide range of devices. In a wind turbine, for instance, magnets in the generator harness motion from the blades and turn it into electricity.
Like many of the other materials needed for clean energy technologies, we can expect a meteoric rise in demand for rare earth metals used in magnets as the world rushes to address climate change.
In the case of neodymium and dysprosium, supply will need to increase sevenfold by 2050 just to meet demand for wind turbines, says Seaver Wang, co-director of the climate and energy team at the Breakthrough Institute, an environment and policy think tank.
In addition, rare earth metal demand for electric vehicles could increase 15-fold from today’s levels by 2040, according to an analysis from the International Energy Agency. And it’s not just clean energy technologies—increased access to electricity and cheap electronics means demand for rare earth metals will rise across other sectors, too.
The world is unlikely to exhaust the geological reserves of rare earth metals anytime soon, Breakthrough's Wang says—rare earth metals aren’t actually all that rare, at least when it comes to the entire planet’s supply. But they don't tend to be very concentrated even in the places they are found, so scaling the supply of rare earth metals quickly and economically enough will be a major challenge.
In the near term, global demand for magnets made with neodymium could triple by 2035, while production will likely only double by then, given the long lead times required to build new mines, according to materials research firm Adamas Intelligence.
Given the growing demand, “the world needs a different solution and technology,” says Jonathan Rowntree, CEO of Niron Magnetics.
Few alternatives to permanent magnets exist today. Recycling can help reduce the need for future rare earth mining and processing, but there won’t be enough used material to meet the growing demand for decades.
Tesla announced in 2023 that it would move away from rare earth metals in its motors in the future, though the company hasn’t shared details about how it will do so. Some experts have speculated that it plans to use lower-powered ferrite materials, which would add bulk and weight to the motor.
Rowntree and his colleagues see iron nitride as part of the solution to the anticipated problem of constraints in the supply of rare earth metals. Iron nitride magnets don’t use those metals, and they don’t require cobalt, another metal sometimes used in magnets (and in lithium-ion batteries) that’s under growing scrutiny because of the environmental and humanitarian issues often associated with its mining. And some experts say these iron-based materials might end up creating magnets just as strong as those that include rare earth metals.
An attractive alternative
Though iron nitride (specifically, a phase called alpha double prime) was discovered in the 1950s, it wasn’t until the 1970s that researchers discovered its strong magnetic properties, says Jian-Ping Wang, a professor at the University of Minnesota and the technical founder and chief scientist at Niron Magnetics.
Even then, scientists couldn’t explain the physics underlying the material’s magnetic properties, and they struggled to recreate magnetic samples reliably through the 1990s. Intrigued by this problem, Wang began work on iron nitride materials at the university in 2002.
After making hundreds of samples and working for nearly a decade, Wang cracked the code to reliably make iron nitride materials in thin films. He presented his findings at a major conference in 2010, the same year geopolitical tensions between Japan and China sparked a huge increase in the price of rare earth metals.
Suddenly, there was a greater appetite for alternatives to rare earths that could be used to make strong permanent magnets. The US Department of Energy’s ARPA-E office sponsored grants to develop such materials, awarding one to Wang and the research that would eventually become Niron Magnetics.
Rare earth metals became ubiquitous across technologies because they represented “a huge jump” in the energy density of magnets when they were discovered in the 1960s, says Matthew Kramer, a senior scientist at Ames National Laboratory.
One of the primary gauges of a magnet’s properties is its energy density, measured in mega-gauss-oersteds (MGOe). While the ferrite magnets on your fridge likely have an MGOe of around 5, neodymium-based magnets are much stronger, reaching around 50 MGOe.
Rare earth metals like neodymium are currently a crucial ingredient in permanent magnets because they can wrangle other metals into an arrangement that helps generate a strong magnetic field.
Permanent magnets produce magnetic fields because of spinning electrons, small charged particles in atoms. Different elements have different numbers of free electrons that in some circumstances can be made to spin in the same direction, generating a magnetic field. The more electrons that are free and spinning in the same direction, the stronger the magnetic field.
Iron has a lot of free electrons, but without an overarching structure they tend to spin in different directions, canceling each other out. Adding in neodymium, dysprosium, and other rare earth metals can help arrange iron atoms in a way that allows their electrons to work together, resulting in powerful magnets.
Iron nitride does what few other materials can: it arranges iron into a structure that gets electrons spinning together in this way and keeps them aligned—no rare earth metals required.
“If you could get the nitrogen to spread these irons out in the appropriate way, you should be able to potentially get a really, really good permanent magnet,” Kramer says. That has proven to be a challenge though, he adds, because it’s difficult to make these materials in bulk and to harness the complex chemistry in a way that forces them to retain their magnetization.
Idea to execution
After Wang was able to reliably create thin films of iron nitride, the next step was to figure out how to make it in bulk, grind it up, and squish it together to make magnets.
Finding a manufacturing process was a challenge in part because iron nitride degrades at high temperatures, which limits the options available in traditional magnet manufacturing, Wang explains. He developed several methods to make iron nitride in bulk, one of the most promising of which involves diffusing nitrogen through iron oxide (rust is a type of iron oxide) under very specific conditions.
In recent years, Niron has focused on perfecting and scaling up the manufacturing process, Rowntree says. A significant remaining challenge is determining how to help iron nitride reach its full potential.
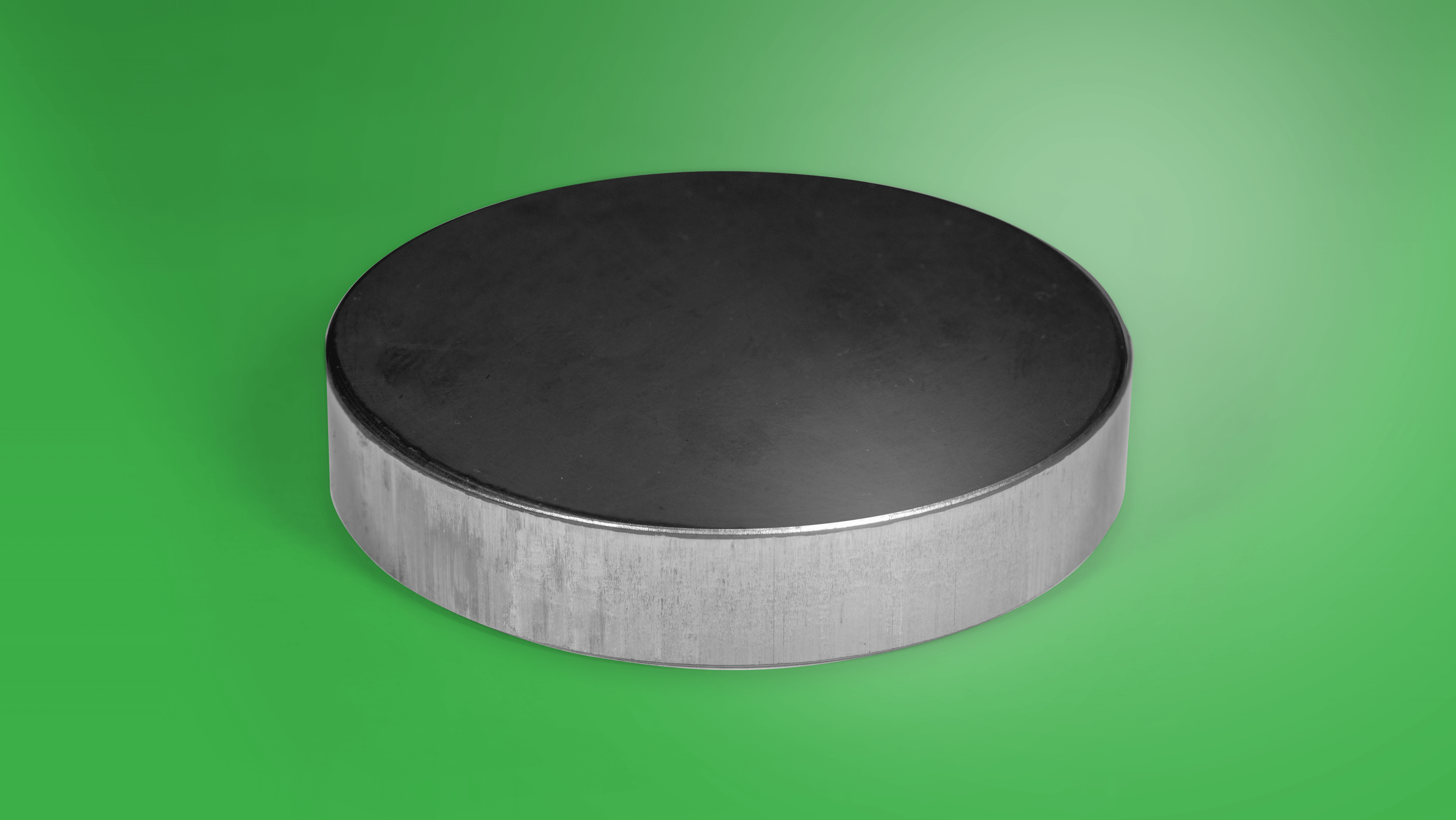
In theory, iron nitride should be able to produce magnets that are even stronger than neodymium ones. But today, Niron’s magnets can only reach around 10 MGOe, Rowntree says. That’s sufficient for devices like speakers, which the company is exploring as an early product. It displayed small speakers made with Niron magnets at CES in January.
With higher magnet strength, iron nitride magnets will be more useful in devices like electric vehicles and wind turbines. In theory, the material should be able to reach 20 to 30 MGOe using Niron’s current manufacturing method, Wang says, though achieving that will require “a lot of optimization.” The theoretical ceiling is much higher, with iron nitride potentially being able to form magnets stronger than the neodymium ones used today.
Niron recently received over $30 million from investors, including GM Ventures and Stellantis Ventures, for a total of more than $100 million in funding. The company is working to scale up production capacity in its current pilot plant, with the aim of reaching 1,000 kilograms of production capacity by the end of 2024.
Niron’s work, along with other alternatives and workarounds, could be crucial in loosening a major potential bottleneck for several critical climate technologies.
“Increased magnets and increased magnet supply are critical to enabling the energy transition,” says Gregg Cremer, an advisor at ARPA-E. “Without more magnets, we’re just not going to be able to meet our objectives.”
Deep Dive
Climate change and energy
The race to get next-generation solar technology on the market
Companies say perovskite tandem solar cells are only a few years from bringing record efficiencies to a solar project near you.
Super-efficient solar cells: 10 Breakthrough Technologies 2024
Solar cells that combine traditional silicon with cutting-edge perovskites could push the efficiency of solar panels to new heights.
Developing climate solutions with green software
Deploying sustainable software practices can reduce emissions and infuse greater efficiency, resiliency, and cost-effectiveness, says director of green software and ecosystems at Intel, Asim Hussain.
Heat pumps: 10 Breakthrough Technologies 2024
Heat pumps are a well-established technology. Now they’re starting to make real progress on decarbonizing homes, buildings, and even manufacturing.
Stay connected
Get the latest updates from
MIT Technology Review
Discover special offers, top stories, upcoming events, and more.